Family SAPINDACEAE
In this article we celebrate bigleaf maple (Acer macrophyllum). Bigleaf maple is neither the tallest nor largest tree species in the watershed, nor the most abundant, economically valuable, nor longest-lived. It is beautiful, but then so are many other species of trees. Perhaps it’s best to say that it has scope, and to tell its story properly requires careful and patient gathering. Here is its unforgettable signature leaf. [Photo 1]
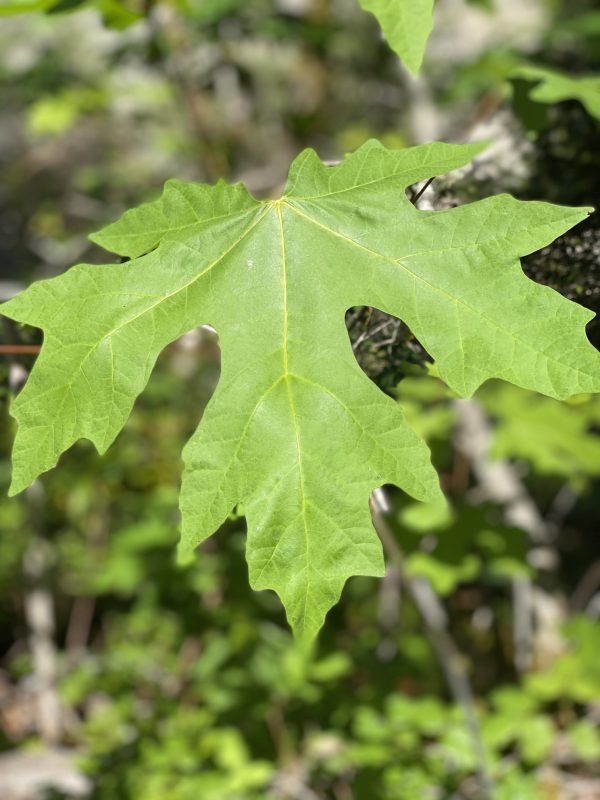
To begin the process of gathering, we locate bigleaf maple in time and place, and then we’ll take a deep dive into its life cycle, a process which is both intriguing and beautiful to behold. We’ll discuss the ecology of the maple and the ecosystem services it provides. Then, we’ll examine some of the ways that human beings have managed to tap into the tree’s abundant and versatile resources.
Introduction
Here in the Gualala watershed you can most easily observe bigleaf maples along Skaggs Springs and Annapolis Roads where the roads cross or wind along the river. The bridge over Haupt Creek on Skaggs Springs Road brings you in close contact with some very nice mature maples. Here is a magnificent maple grove fully leafed out located along the Wheatfield Fork and visible from the bridge at Valley Crossing. [Photo 2]
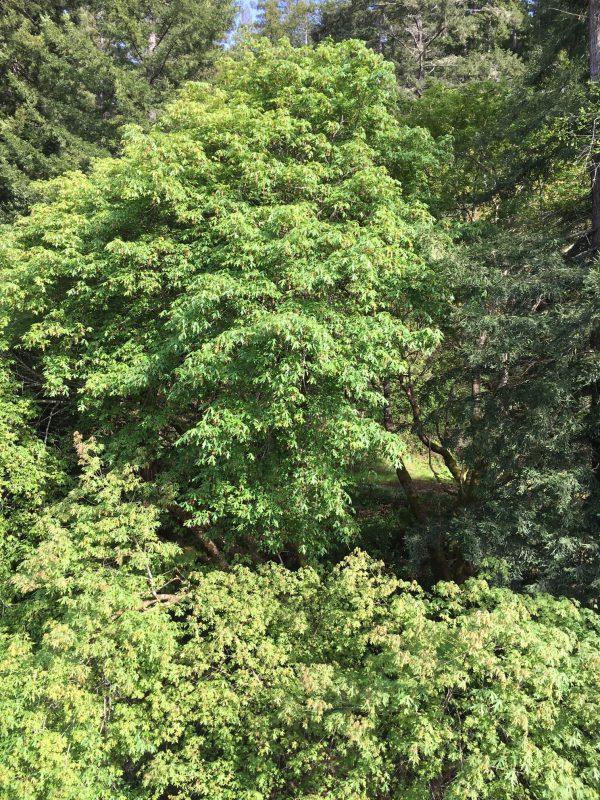
Maples also occur where springs or seeps create moist conditions, though they can occur even in drier locations like talus slopes. In the fall the leaves turn a lemony yellow and light up canyons, making them easy to pick out. The scientific name of the species is macrophyllum which means big leaf and indeed the palmate leaves can grow to 12 inches or more in width. [Photo 3]
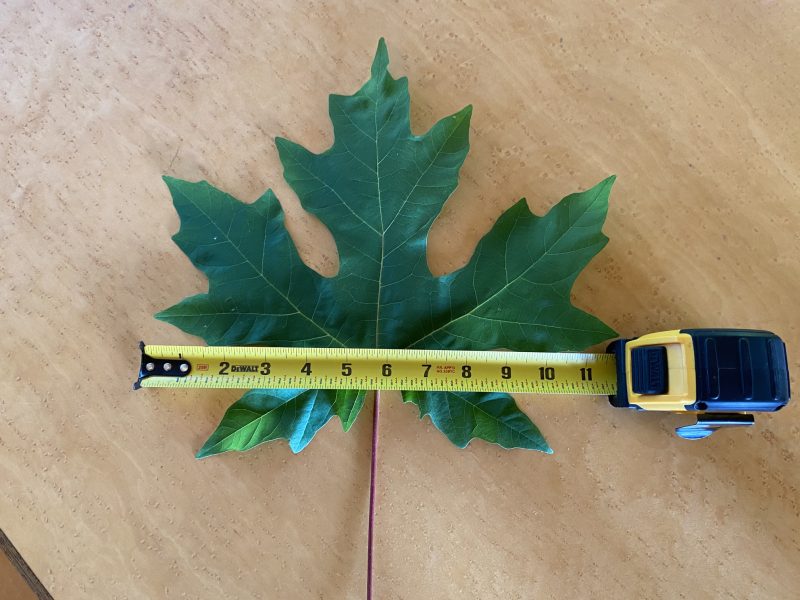
Bigleaf maple occurs along the coastal Pacific Northwest [Figure 1] from California to British Columbia and ranges into the western slope of the Sierra Nevada. Recent research suggests that it most likely evolved from maples in East Asia, migrating across the Bering Land Bridge during the Miocene, millions of years before humans evolved. It is the largest of the dozen maple species in North America and the only tree-sized maple in California.
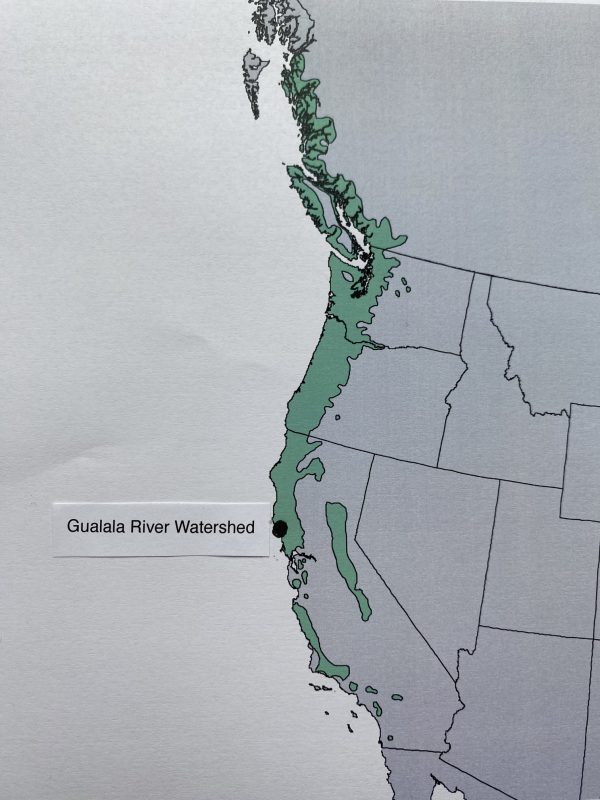
The official California state champion maple tree is located in Humboldt County on the campus of the Mattole Valley School and tops out at 76 feet in height and a whopping 18 feet in circumference. [Photos 4 and 5]
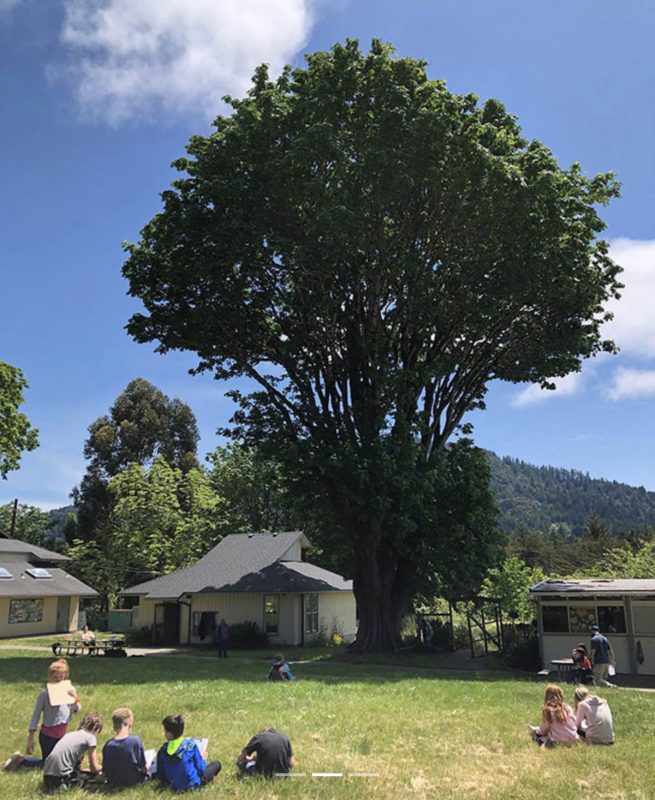
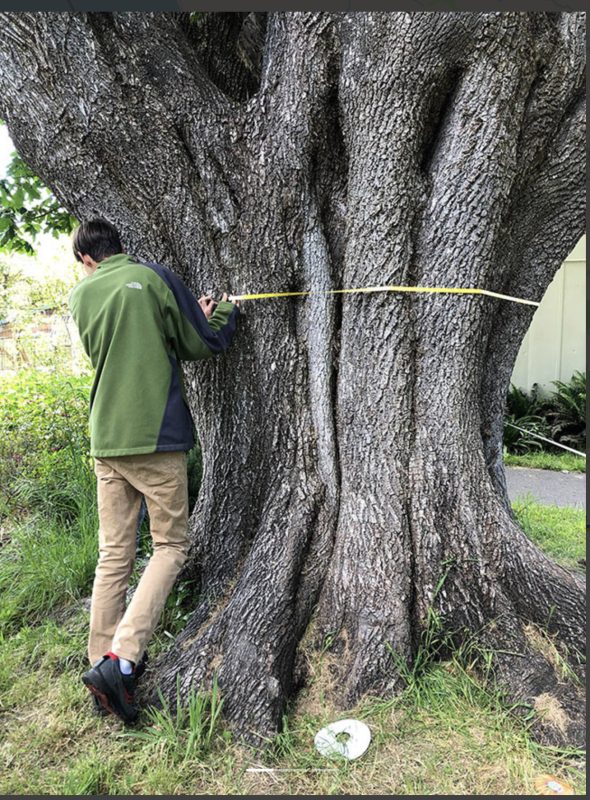
This tree is very old—perhaps 200 years or so. It began producing flowers and seeds at around 10 years of age, perhaps some time in the early 1800s. It could live another hundred years. The maples we encounter in the Gualala River watershed are smaller, commonly up to 40 feet or so in height but with broad canopies where space allows.
Focusing on Phenology: Buds, Flowers, and Leaves
Each year, in the span of 6 months from March through October, the bigleaf maple passes through a series of crucial events: from budding and bud burst to flowering, leafing out, pollinating, fruiting, changing leaf color to leaf drop. Together these events make up the tree’s phenology— the timed response of plants to the cues of natural conditions like day length, temperature, rainfall, etc. Botanists and climate scientists are keen to study these cyclic stages in different species and what effect climate change will have upon them. Bigleaf maple is one of the species tracked in a nationwide citizen science project of the Chicago Botanic Garden. One of the distinct advantages of observing bigleaf maple structures is that they are large and easy to see. Figure 2 shows the form used by the Garden’s Budburst program to record the various phenological events.
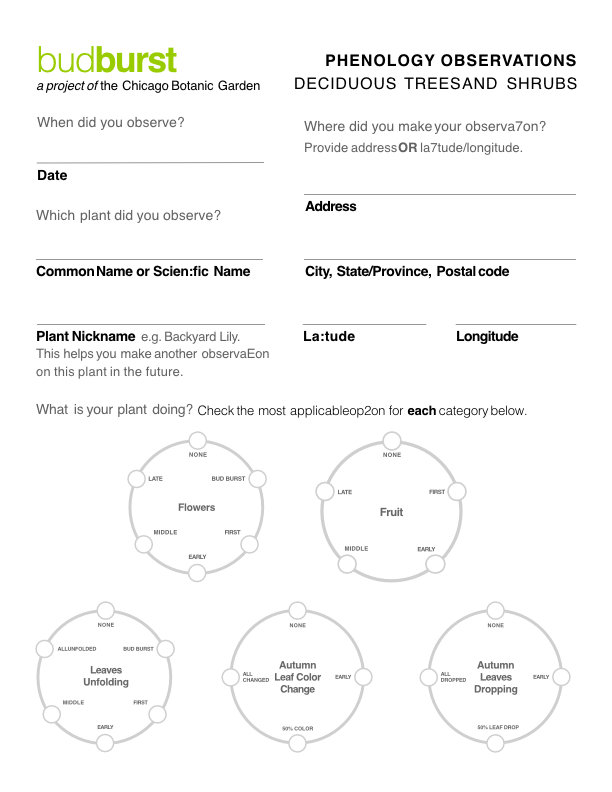
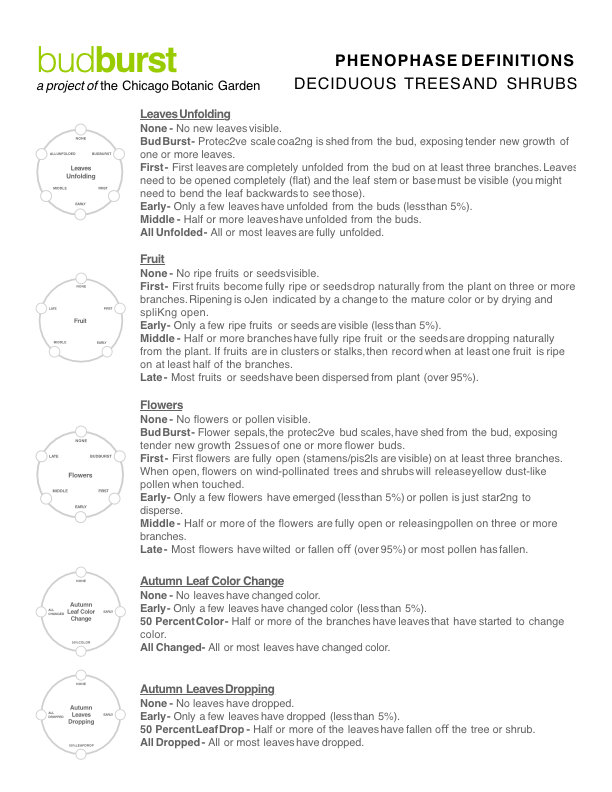
For our purposes we track these stages or phenophases closely through a series of photos. The annual phenological clock begins with the observation of buds. In early spring, the primordial flowers and leaves begin growing within fat, tightly closed buds at the tips and sides of the branches. [Photos 6 and 7]
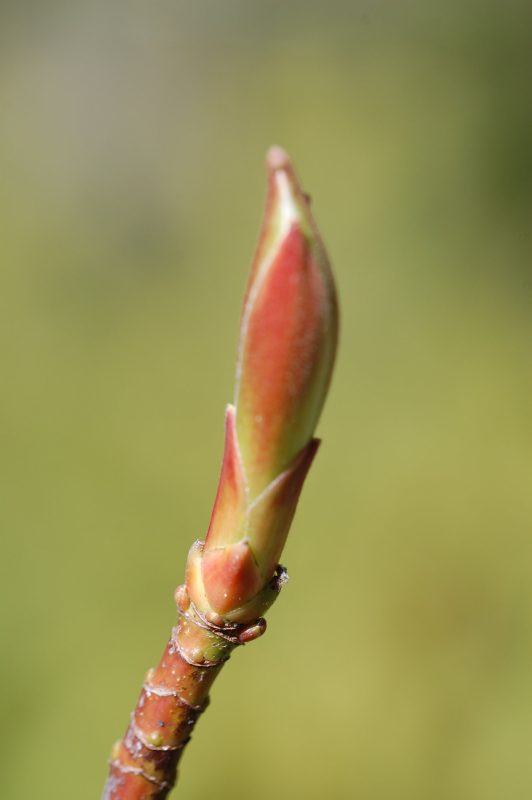
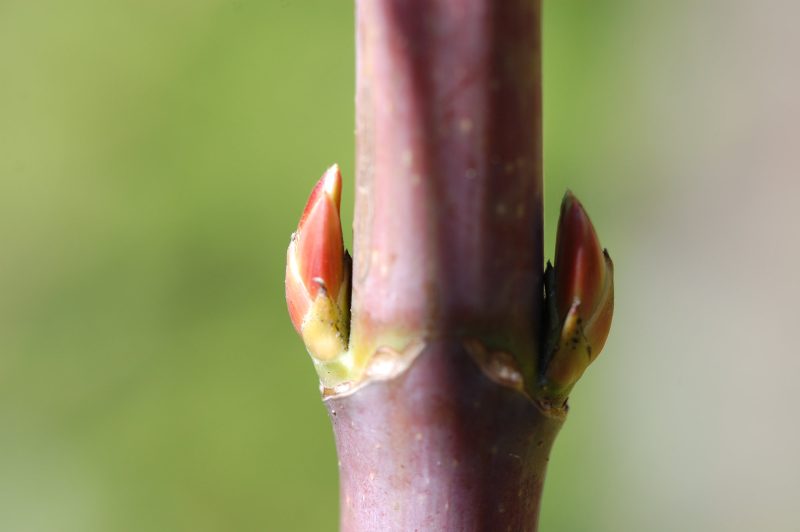
These buds formed with energy stored at the end of the growing season last year. They remained dormant throughout the winter. But more daylight and rising temperatures have now cued the buds to resume growing so that the leaves can unfurl in time to take advantage of the abundant sunlight and thereby maximize their period of growth.
Here’s a terminal bud (one that occurs at the end of a branch) in early spring. [Photo 8]
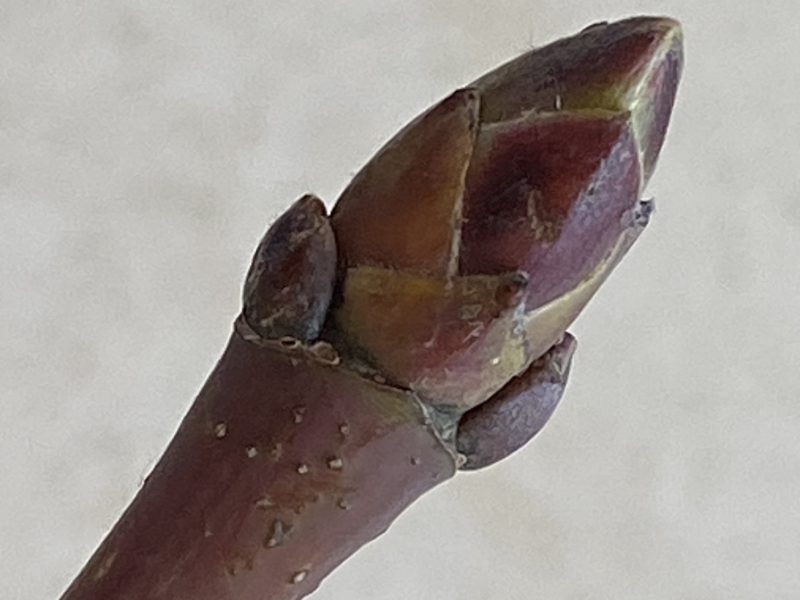
The tough outer scales of the bud protect the tender tissues inside. And here’s the same bud after being cut open to reveal the pale tissue of a structure called the apical meristem which will develop into flowers and leaves. At the very center the textured yellow tissues are the developing (primordial) flowers. [Photo 9]
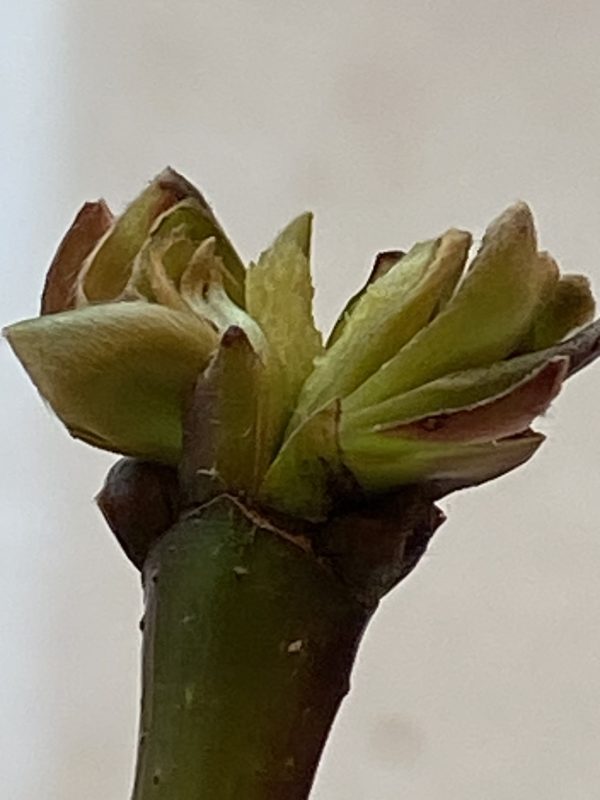
In just a few weeks, the buds open during a stage called bud burst which typically happens here in our local maples in April. This series of photos displays the changes that happen from bud burst through the emergence of flowers and leaves. [Photos 10, 11, 12, 13, 14, 15, 16, 17]
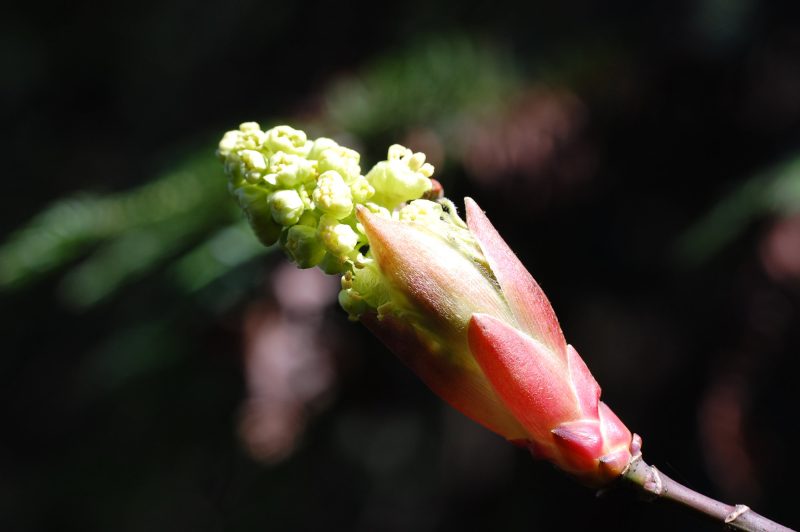
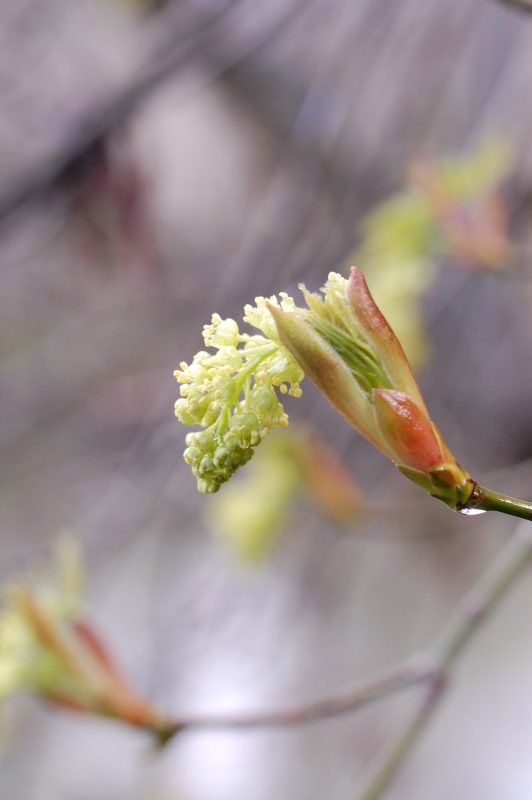
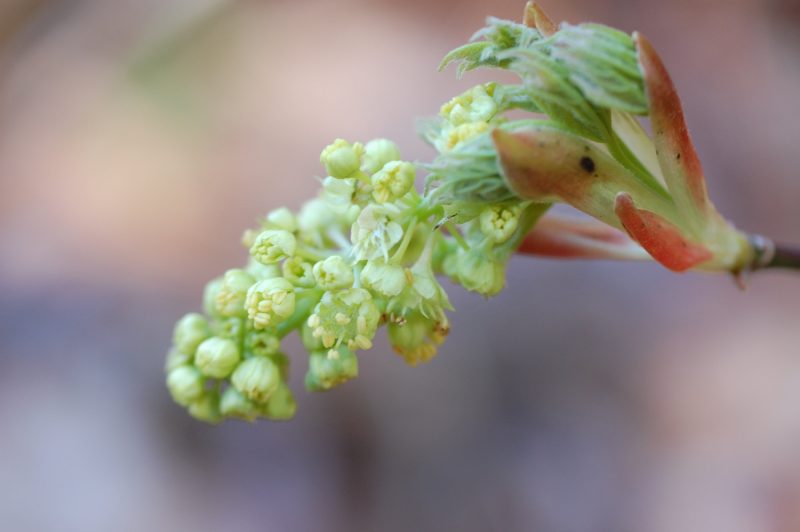
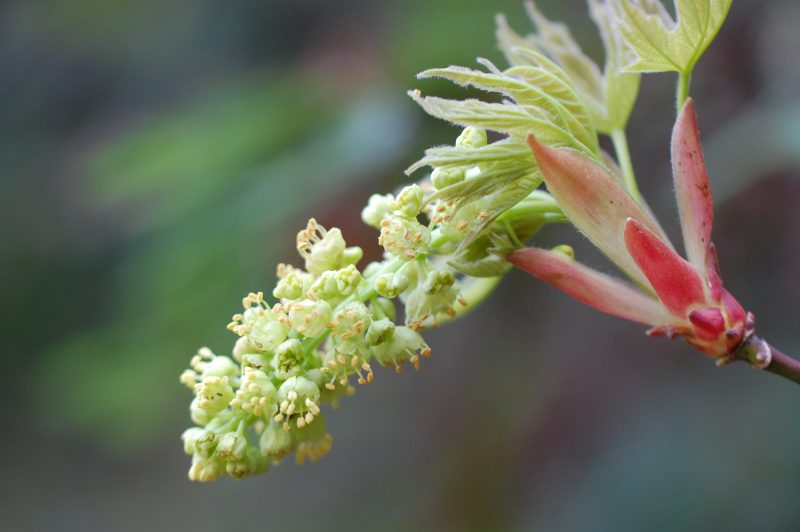
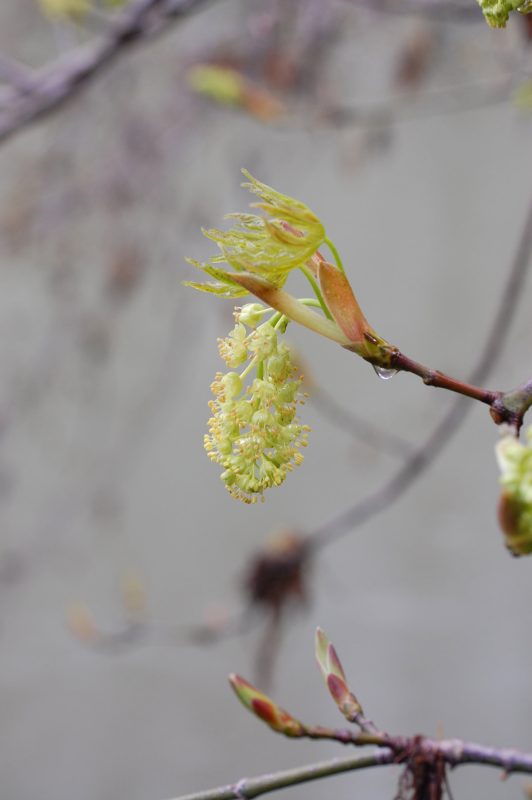
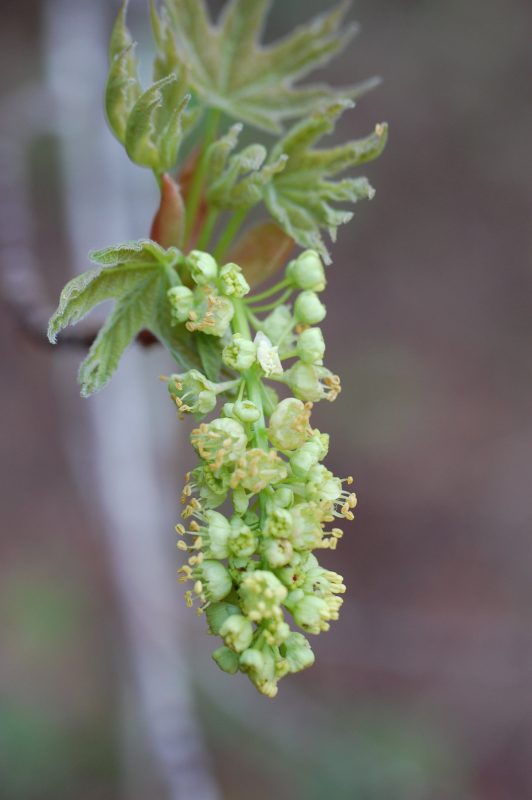
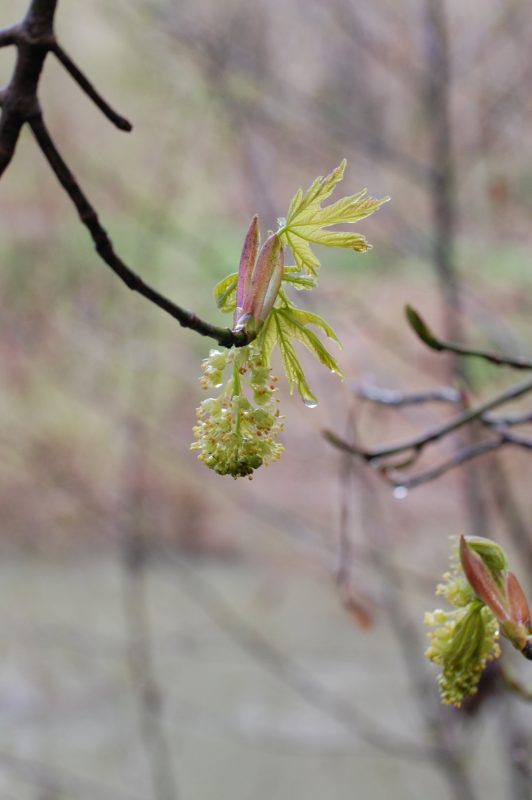
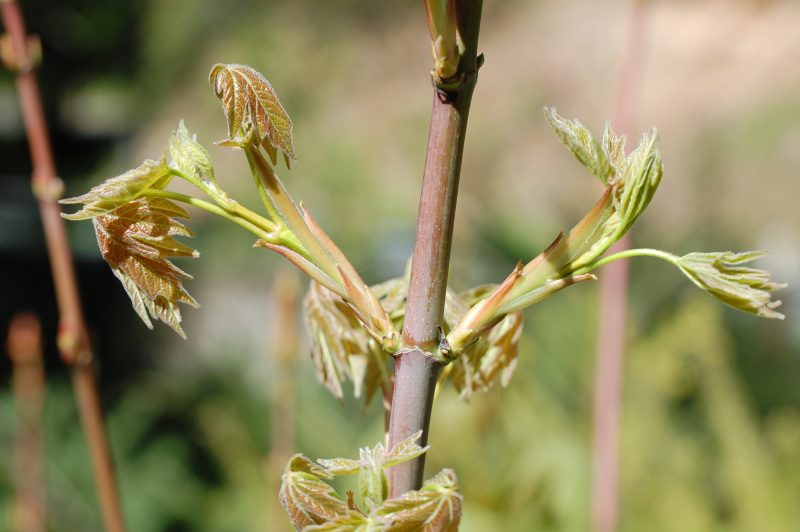
As the flowers emerge, many insects are attracted to the sweet-smelling nectar and pollen which provide them an early season bounty. The insects return the favor as they pollinate the flowers in the process. [Photos 18, 19, 20]
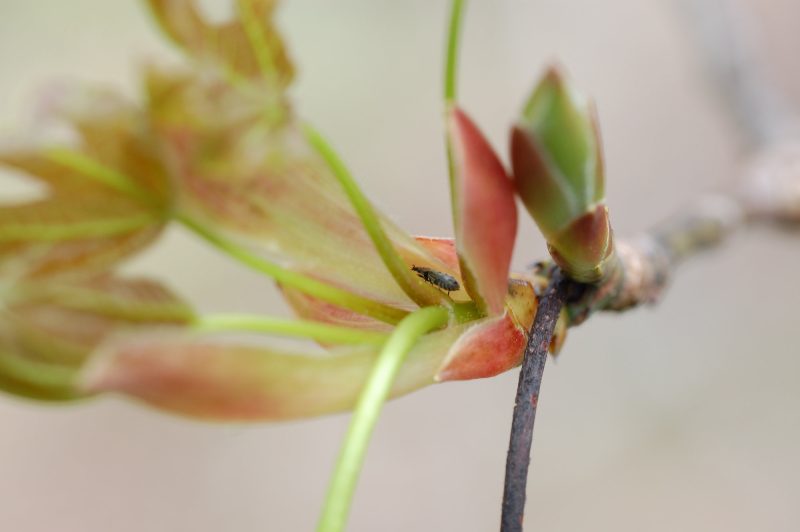
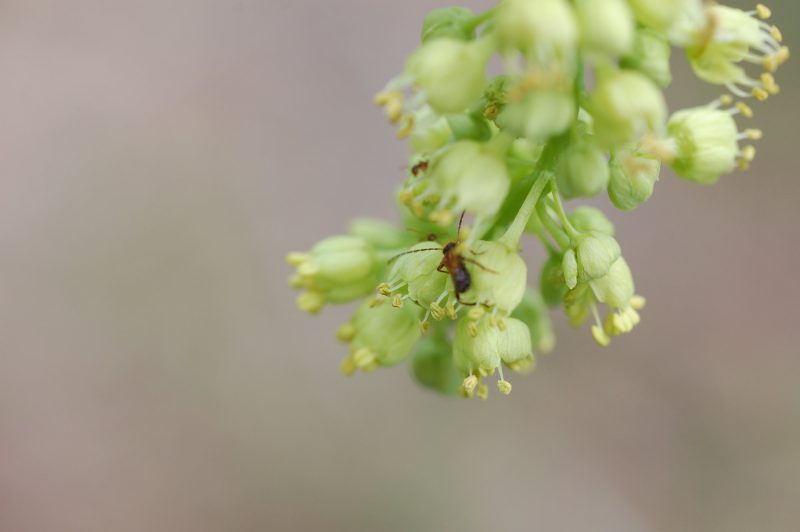
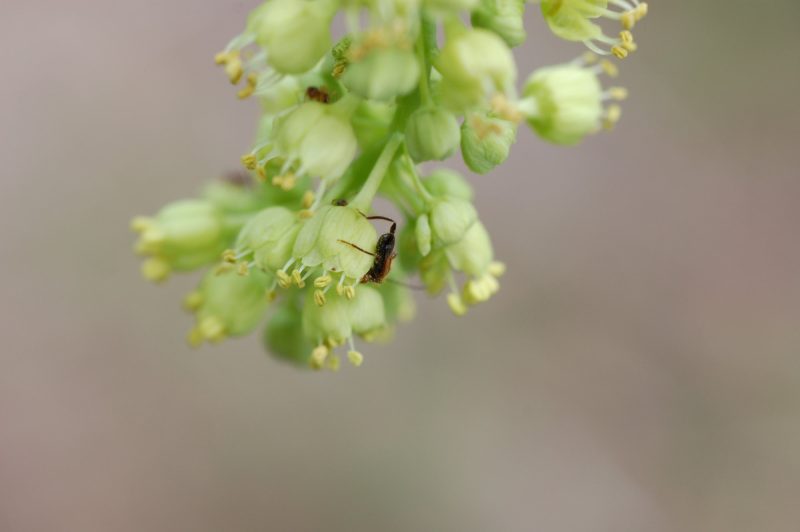
The insects depend upon the nectar and pollen and the tree depends upon the pollination services of the insects. Timing is everything, and climate could change timing in such a way that the insects and flowers don’t meet.
As new leaves begin to unfurl, fluids circulate through the conductive tissue of the xylem in the veins of the leaves. Tender developing parts such as leaves and petioles (the stalks that connect the leaves to the branches) are initially colored red, perhaps with a pigment to protect them from damaging radiation. [Photo 21]
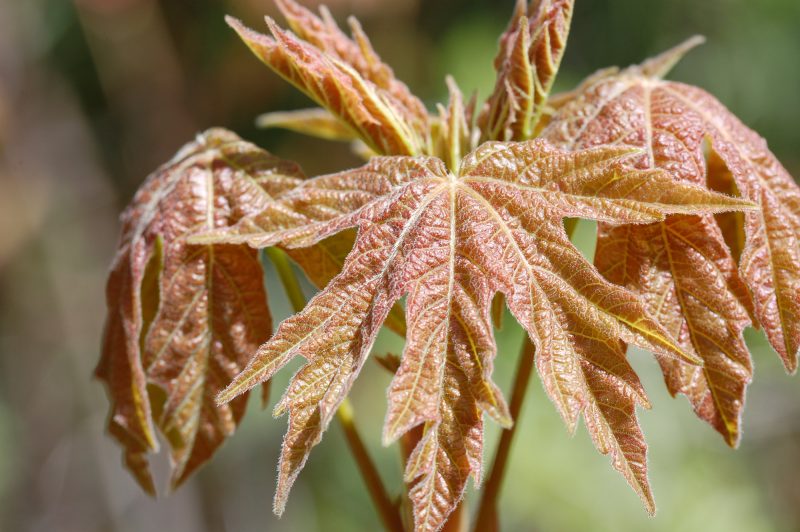
Leaves as Solar Collectors, Food Producers, and Power Plants
Maple leaves remain on the tree until late fall. During this period they play a vital role in a tree’s life as the factories for many essential processes. First and foremost, they are living solar collectors, among the largest in North America. As such, they capture the energy of sunlight and convert it to chemical energy in the form of sugars during the process of photosynthesis, one of the most important biochemical processes on earth.
PHOTOSYNTHESIS
carbon dioxide + water + light energy = glucose + oxygen
Can you imagine making your own food with just your body, as maples do? As the only organisms that can capture sunlight, green plants are the primary producers and the basis for the rest of the planetary food web.
Photosynthesis takes place within microscopic structures in the leaves called chloroplasts. These contain the pigment chlorophyll which absorbs the red and blue portions of the visible spectrum and reflects the green wavelengths which are not absorbed—the reason why the leaves look green. Chloroplasts and stomata are too small to see individually with the naked eye, but groups of active chloroplasts are visible as green lines running along the veins of the new maple leaves. [Photo 22]
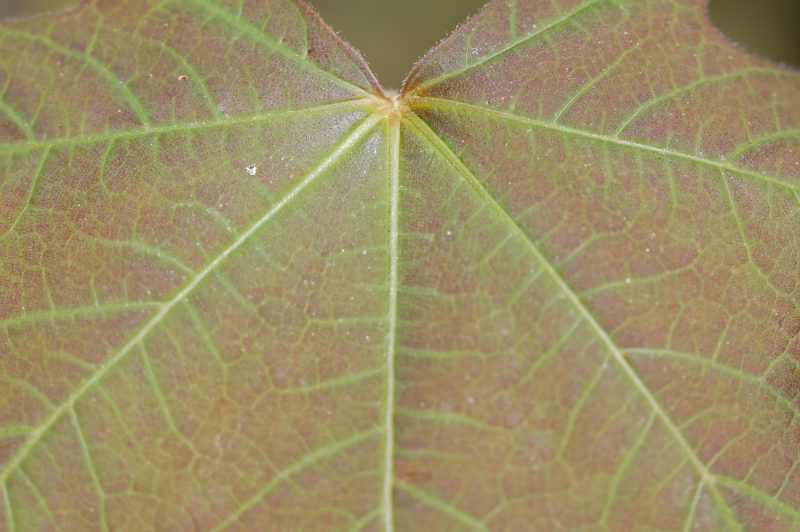
Trees don’t possess lungs, but gases are exchanged in the leaves through small openings or pores called stomata (little mouths) which open and close to admit carbon dioxide and water vapor and release oxygen. The stomata can also release water vapor in a process called transpiration in which water is drawn up from the roots and throughout the tree and expelled through the leaves, supporting the tree’s hydraulics or circulation. This process cools the leaves and moistens the air around the maple tree and together with the shade that the leaves produce makes a comfortable climate-controlled environment for wildlife and humans alike. Perhaps most important of all, the oxygen that green plants release is a huge source of the atmospheric oxygen that enables terrestrial life to survive and flourish. Until green plants evolved and created an atmosphere rich in oxygen, terrestrial life was impossible.
As the spring and summer proceed, it’s possible to observe the many ways in which the structures of the maple tree formed to maximize the interception of sunlight. The leaf petioles and their attachment to the branches maintain the exposure of the leaves to sunlight with as little overlap as possible. The leaves are attached in opposite pairs to the branches via very long petioles that hold the leaves away from the branch and each other. [Photos 23, 24]
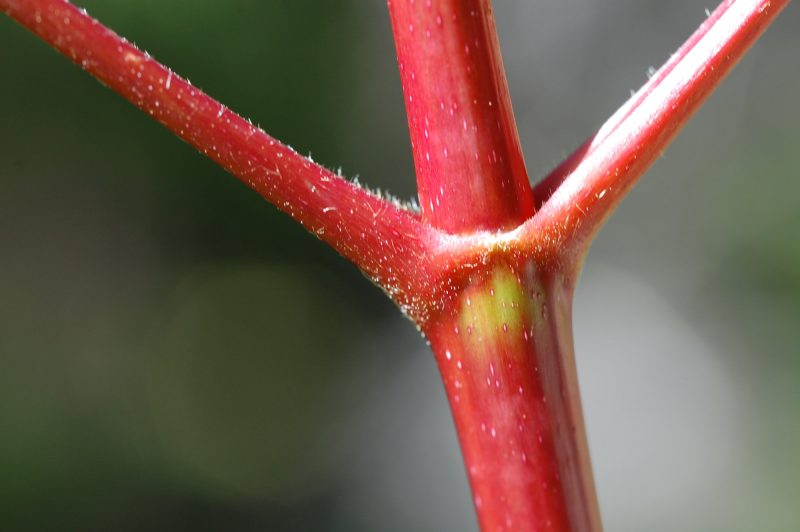
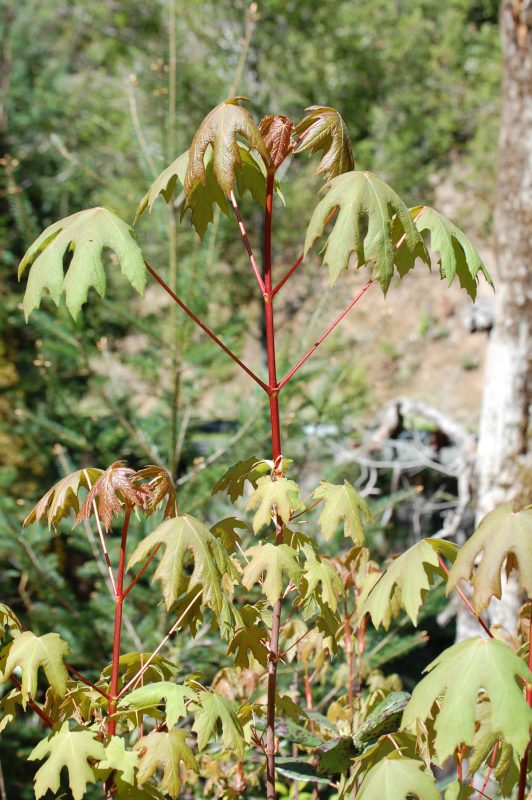
Each pair alternates the place of the attachment to the branch so that the leaves stand out like mini parasols. [Photo 25]
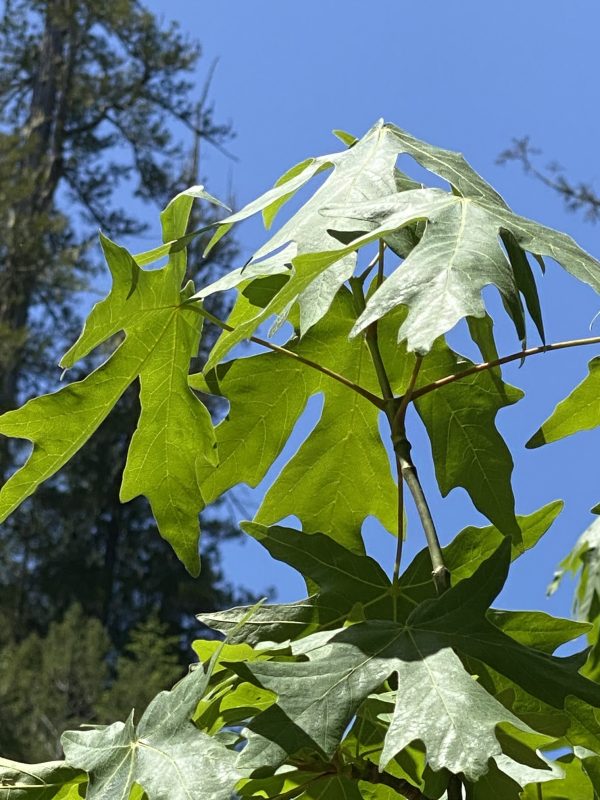
During the growing season, leaves of the maple account for the largest surface area of any portion of the tree, collectively intercepting so much sunlight that beneath the tree there is deep shade. [Photos 26 and 27]
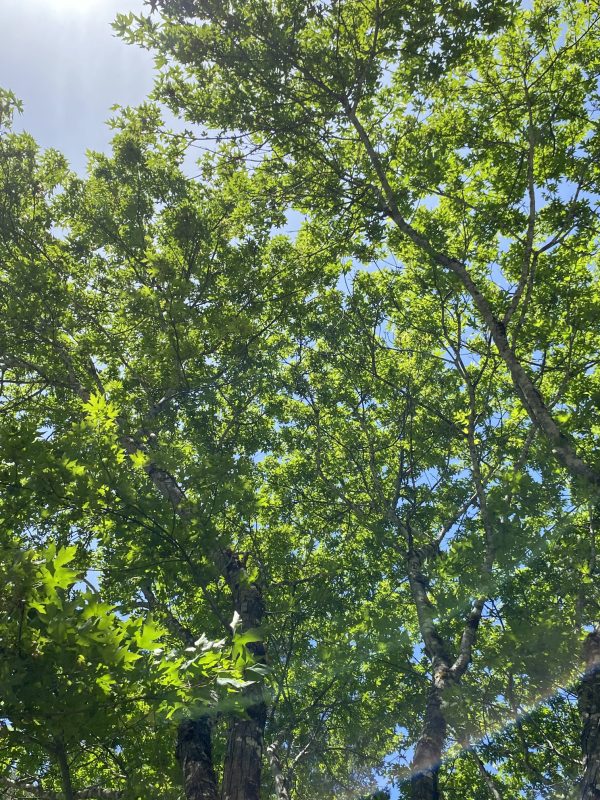
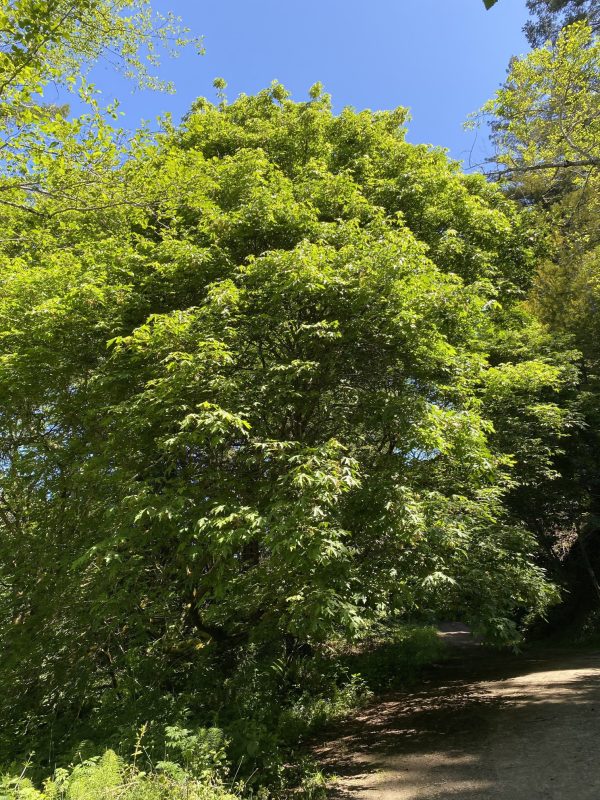
Notice how the scaffolding of the tree spreads the branches out in all directions. [Photo 28].
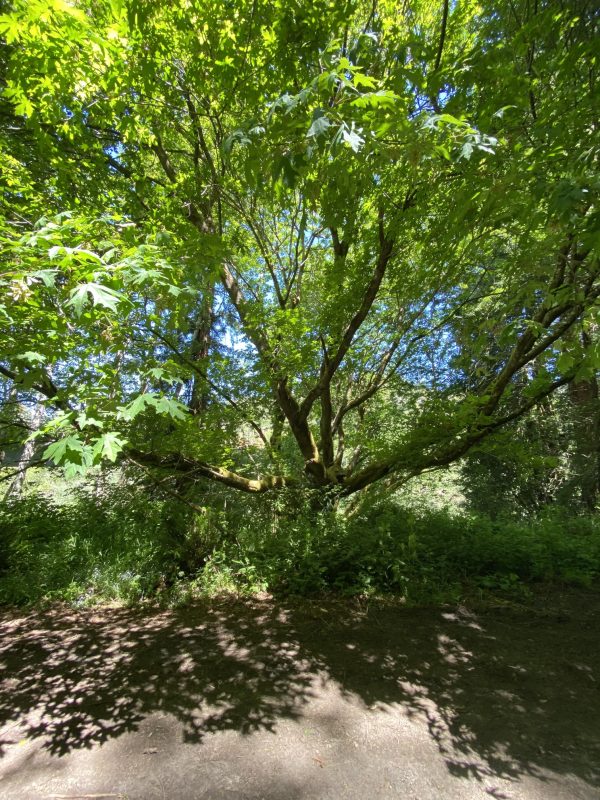
Remember when the racemes of flowers were first emerging, the leaves were positioned above them in Photos 12-16? In May as the flowers develop into 2-winged fruits (samaras), they are positioned beneath the large leaves where they are protected from intense solar radiation and also don’t block sunlight to the leaves. [Photo 29]
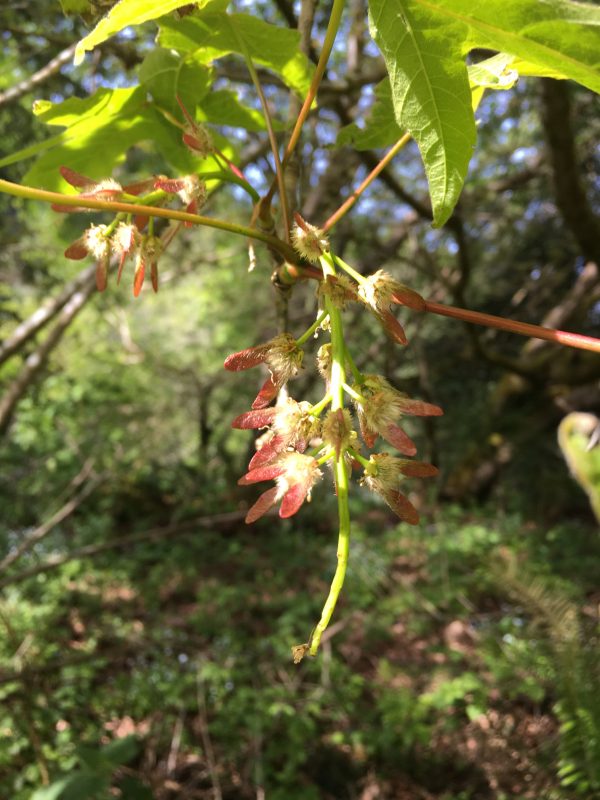
Now the tree as power plant swings into full production, with the photosynthesizing leaves providing energy to the samaras as well as to all the rest of the tree parts. [Photo 30]
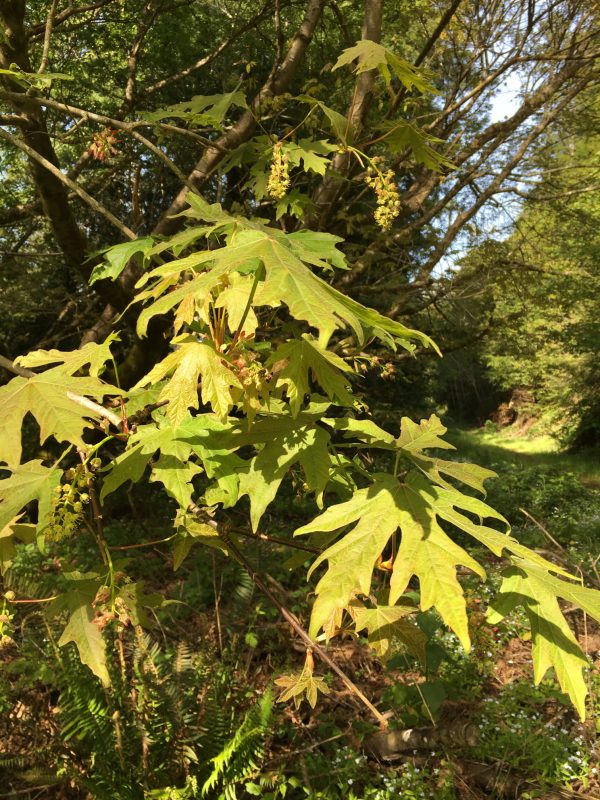
Fruits, Samaras, Whirligigs
Samaras, too, have their adaptations—their role is to protect the developing seeds and then disperse them as widely as possible. They have bristly hairs that are thought to discourage animals from eating them, though squirrels, wood rats, and birds gobble up the seeds inside of them. [Photo 31]
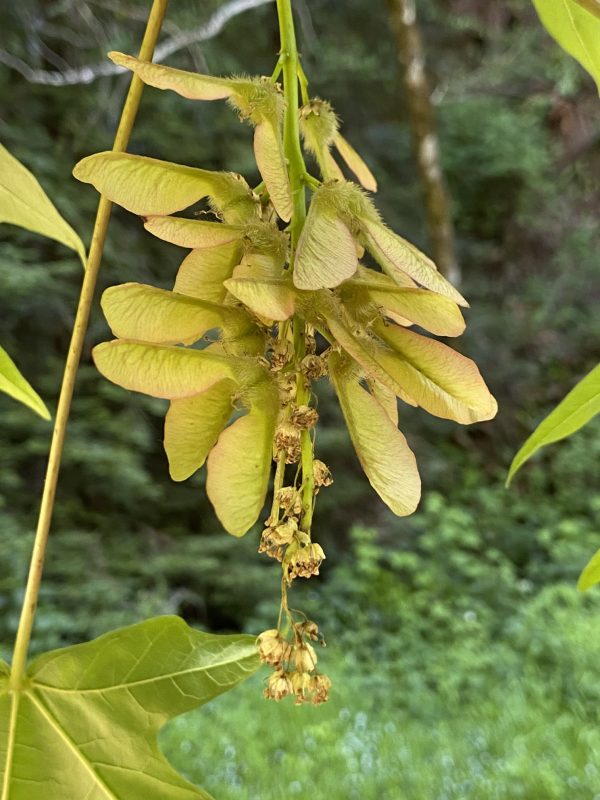
In late summer the two wings of the samaras split apart. Breezes help the samaras detach from the branch and, like whirligigs or helicopters, they spin down to the ground. The spiral flight slows the descent of the samara as an adaptation to catch wind and disperse the seed a greater distance than if it simply dropped directly with gravity. Curious children and NASA space engineers have studied the flight of maple samaras over the years, but it wasn’t until 2009 that a team of scientists “elucidated the aerodynamic mechanism for high lift in autorotating seeds.” That’s science speak for, “the shape of the samara creates lift as it spins down through the air.” On the ground, the outer coat of the samara decomposes, releasing the seed into the rich forest litter until mid-winter rains trigger its germination into a seedling. [Photo 32]
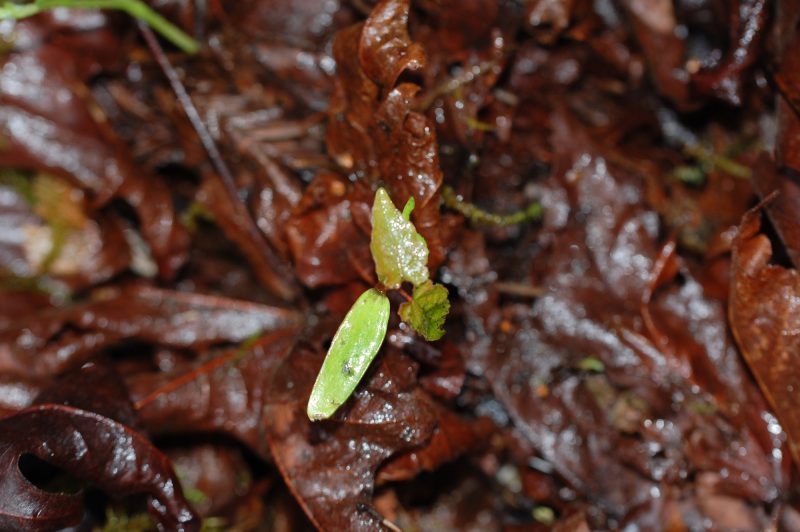
Color Change and Leaf Drop
As days shorten and temperatures begin to drop in the fall after the equinox, the tree responds with changes in the internal chemistry in the leaves. The chlorophyll breaks down, revealing the characteristic yellow carotene pigment in the leaves. [Photo 33]
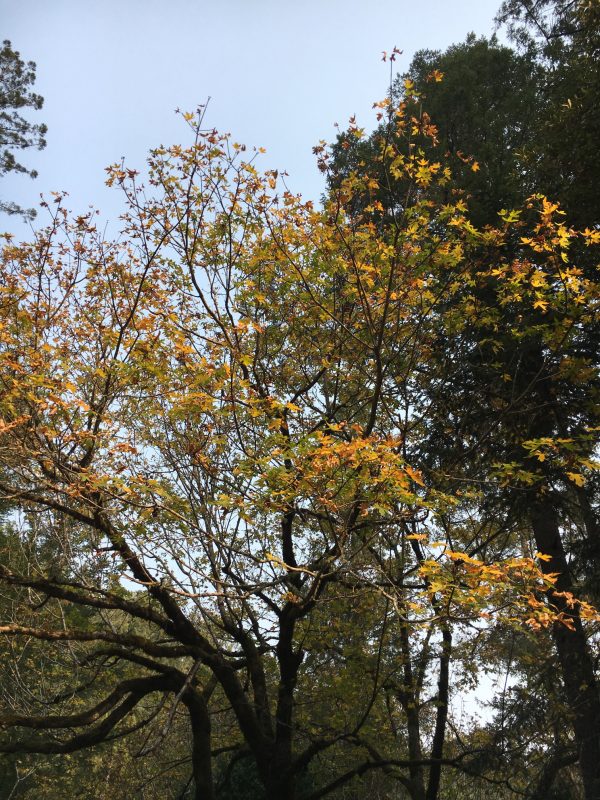
Here on the West Coast bigleaf maples provide perhaps our most beautiful display of fall color. This video by Scientific American gives an excellent explanation of changing leaf color. Please note that the video emphasizes trees in the eastern U.S. where fall color displays are spectacular. Bigleaf maple doesn’t grow there, but it does undergo the same process.
In late October, leaf drop begins with a process called abscission. At the base of the leaf petiole where it connects to the branch, there is a line of cells that swell and burst under the influence of hormones, severing the connection of the leaf to the branch, and the leaves fall. The end of the phenological observation for the year comes with the fall of the last leaf. In bigleaf maple the rich organic leaf litter beneath the trees decomposes rapidly, making nitrogen and other nutrients available to the tree and to understory plants. [Photo 34]
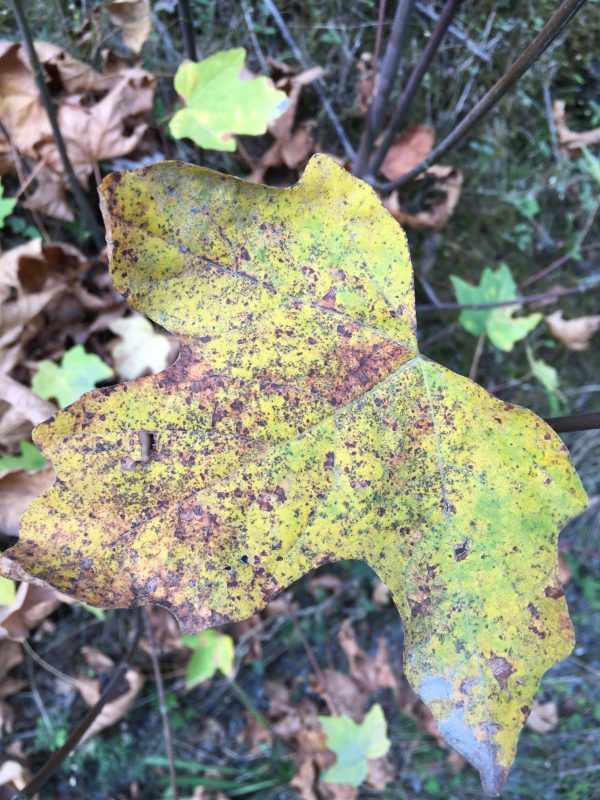
Ecology and Ecosystem Services
Ecosystem Services
Bigleaf maple is often a dominant or co-dominant tree with other tree species. Along the riparian corridor, it usually occurs with alders, redwoods, and Douglas fir, most often slightly upslope of alders along the river bank. Unlike alder, it does not do well for very long periods in standing water, but it can tolerate some flooding.
In the riparian hardwood forest, bigleaf maple contributes a number of ecosystem services to the environment around it. Maple trees help stabilize banks, filter pollutants from runoff, and cool the soil with their shade. The flowers provide nectar for insects in the spring, and the seeds and dense foliage are prized by wood rats and birds for food and nesting sites. Deer browse on saplings (and can do considerable damage to them in the process). And at the end of the growing season, fallen leaves decompose rapidly into rich humus that helps fertilize the forest. These services are just some of the ones that we know about. The more we study trees, the more we learn how valuable they are to the ecosystems where they grow. And while it’s impossible to assign a monetary value to the services they provide, it’s abundantly clear that they are a large part of the natural capital in a watershed, helping to hold life together.
Epiphytic Community
In winter with the leaves gone, the maple reveals its epiphytic community of ferns, mosses, liverworts, and lichens which appear like velvety green sleeves on the branches and trunk. [Photos 35, 36]
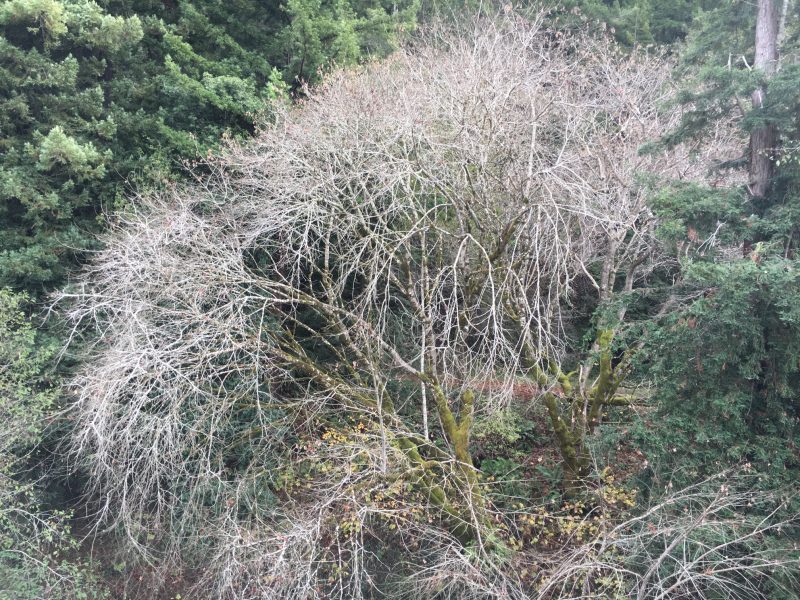
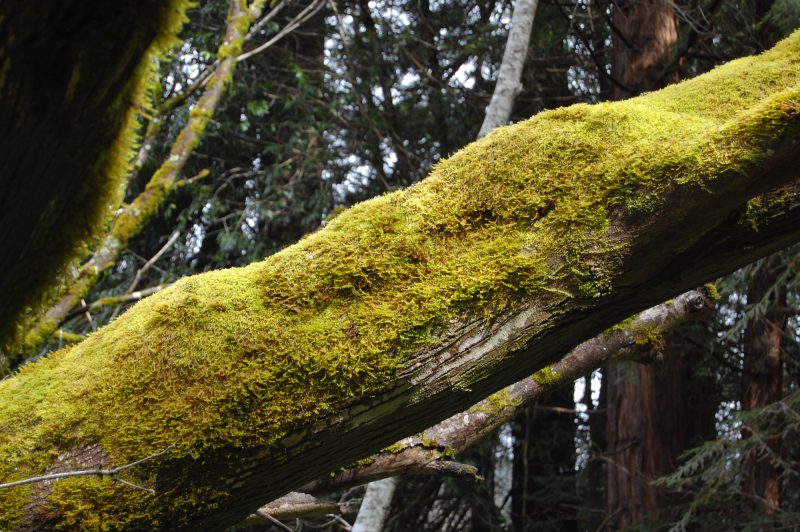
Calcium in the bark provides a substrate for calcium-loving epiphytes. Winter light filters down to the trunk and branches and stimulates the process of photosynthesis in these small plants and lichens. In the temperate rainforests of Washington, this associated plant community can grow up to a foot deep and provide up to a ton per tree of biomass. As the epiphytic community builds up over time, it creates canopy soil which accumulates in the crevices of the bark and crotches of branches. Canopy soil is a rich blend of nutrients available to the tree which can tap into this suspended soil with adventitious (aerial) roots. Thus, while the tree provides the epiphytes with a place to grow, they in turn provide nutrients for the tree and improve the humus in the litter benefitting other nearby tree species, especially conifers—yet another example of the exchange of ecosystem services. Here in our watershed, the epiphytic community is active though more modest, given lower rates of rainfall.
Fungal Associates
Recent research has shown that the fungal community associated with canopy soil in the trees differs from that on the ground, adding to the biodiversity of the community. The fungal associates of bigleaf maple roots are arbuscular, occurring inside the root tips where they facilitate the exchange of water and nutrients for the tree’s sugars. Experienced foragers in the Gualala River watershed know that they are unlikely to find mushrooms under the maples since the arbuscular fungi don’t produce mushrooms.
Legal Standing and Protection
In California, the Department of Fish and Wildlife designates the Bigleaf Maple (Acer macrophyllum) Alliance as sensitive and rare. An alliance is a vegetation community established by the state’s Vegetation Classification and Mapping Program and rated for its degree of rarity and level of threat for conservation purposes. While bigleaf maple itself is not rare, the plant community in which it is dominant is sensitive and rare and therefore falls under the protection of the California Environmental Quality Act. Sonoma County vegetation has been recently mapped and classified and the results published in a report in 2015. [Detailed information on the alliance and associated plants is available in Classification of the Vegetation Alliances and Associations of Sonoma County, California, Volume 2 of 2 – Vegetation Descriptions.] The portion of the Gualala River watershed that lies in Mendocino County was not mapped for this project.
The Many Uses of Maple
In this series on trees, we don’t usually go into depth about the human uses of trees, preferring to focus on their intrinsic value as part of wild ecosystems. But maple is a versatile and valuable economic resource, and we humans are a clever species capable of experimenting and extracting multiple diverse products from the trees around us. The gifts of maple appeal in particular to the human senses.
People often associate the word maple with syrup (and waffles and pancakes!). Most of the commercial production of maple syrup comes from the sugar maple (Acer saccarhum) which grows in the Eastern hardwood forests. It’s possible to make syrup from the sap of bigleaf maples; however, the sugar content of bigleaf maple sap is not as high as it is in sugar maple so it takes a lot more sap to make syrup from bigleaf maple trees. But people do.
Maple also makes beautiful furniture. Among the most prized types of maple wood are those with “figure.” Terms such as curly, flame, quilted, bird’s eye, and spalting all refer to patterns of growth that differ from normal growth. When worked, wood with figure comes alive in beautiful lustrous detail. The wood database provides detailed information about many different woods. And there are excellent videos online that highlight the qualities of bigleaf maple for those interested in working with it.
Maple has a voice…
One of the most important but less widely known uses of maple is as tone wood—wood that is used to create soundboards and other parts of stringed instruments. Luthiers—those skilled craftsmen who make stringed instruments like violins, cellos, guitars, etc.—love maple. When strings are plucked or bowed, the vibrations make sounds, but the sounds are faint and require a soundboard to amplify them. The quality of the wood in a soundboard makes a big difference in the sound, and maple (including bigleaf maple) has just the right combination of density, strength, shock-resistance, hardness, grain, plus beauty that makes it ideal for soundboards.
Luthiers wanting to re-create the beautiful, rich sound of Stradivarius violins have spent centuries investigating Stradivari’s craft—the exact proportions of each piece, how to properly age the wood, the mineral and chemical treatments of the wood, and of course, the source of the wood! In his workshop in Cremona, Italy in the late 1600s and early 1700s, Stradivari crafted over 1100 instruments using maple species (Acer pseudoplatanus and A. platanoides) from the mountains of the regions of what today are Serbia, Bosnia, and Croatia. [Photos 37, 38]
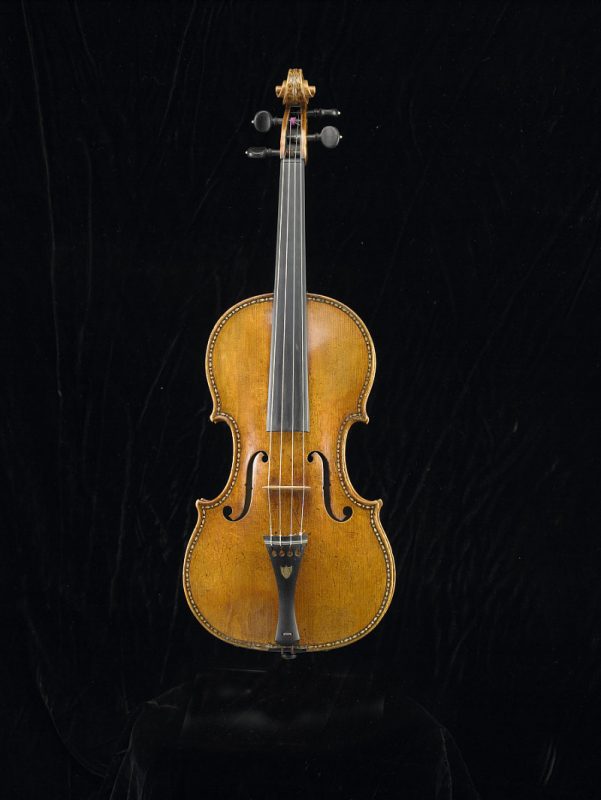
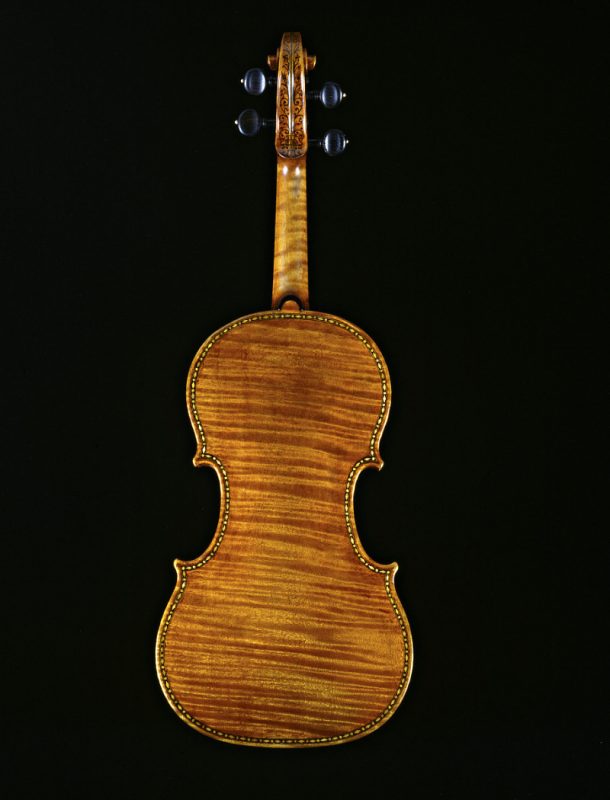
The best wood came from large, old-growth trees harvested in winter. Was there anything special about those trees? There has been speculation that the consistent size of growth rings (both early and late wood) produced by the unusual cold of that time might have produced the ideal wood. Europe was undergoing the Little Ice Age (the Maunder Minimum)—a climate anomaly during which temperatures plunged—and the normally thicker, spongier rings of early wood produced during the growing season were consequently narrow, and more closely matched those of late wood. Could the climate-induced changes in the wood account for the secret of the rich sound of Stradivarius violins? Other research suggests that it might be the special baths and treatments of the wood that the master craftsman employed. What is indisputable is that maple was his wood of choice for the soundboard (back) of the instrument as well as for the neck and sides.
While the international tone wood trade allows for a much wider choice of woods than in the 1700s (today’s woods include those from the tropics like mahogany, rosewood and koa–much of which has been over harvested), North American luthiers continue to include bigleaf maple in their stock of woods. (For a fascinating account of the creation of violins and cellos from tree cut to finished product, visit this luthier’s site). But choice maple tone wood comes from increasingly rare large old-growth trees that grow figured wood and which have been left in the forest after logging operations. Availability is inevitably linked to sustainability. At least one company has opted to focus on growing its own bigleaf maple tone wood rather than harvesting it from the wild where market forces put sustainability at risk.
Chances are, if you’ve listened to music today, you’ve heard maple playing a key part. Here is Joshua Bell with the Baltimore Symphony Orchestra playing an excerpt from the score of the movie, The Red Violin, on his 3 centuries’ old Stradivarius violin. Composer John Corigliano’s dramatic score shows off the astonishing range of the violin and the superb playing of the young virtuoso Bell. Take 5 and listen to the spectacular sounds that Bell and the orchestra are able to make from strings and wood, much of it maple—of course along with the winds, horns, and percussion.
Will bigleaf maple last?
The pleasures of bigleaf maple are many. We have relied upon its finely tuned phenology to deliver these gifts without understanding or reflecting upon what makes them possible. We know now that trees are sensitive indicators of climate change. Since 2010 in the state of Washington where bigleaf maples reach some of their finest and most expressive specimens, maples have shown signs of severe stress defined as “loss of vigor, loss of transpiration, and reduced photosynthesis due to leaf loss”. Many trees have died. Recent research reveals that extreme heat, especially along roads and areas of development, have pushed the trees past their limits of temperature tolerance. It is beyond ironic that these trees which are among the most popular shade trees along the Pacific coast may no longer be able to cool us because we have overheated them. Are we too late or can we, the clever species, restore the balance for them and for us?
BIBLIOGRAPHY
Betzen, Jacob J. et al. Bigleaf maple, Acer macrophyllum Pursh, decline in western Washington, USA. Forest Ecology and Management. Vol. 501. December 01, 2021, 119681.
California Native Plant Society. A Manual of California Vegetation.
Cantor, Cliff. 9/29/2017. Maple Seeds: Nature’s Helicopters. Trees Pacific NW.
Fryer, Janet L. 2011. Acer macrophyllum, big leaf maple. In: Fire Effects Information System, [Online]. U.S. Department of Agriculture, Forest Service, Rocky Mountain Research Station, Fire Sciences Laboratory (Producer).
Jian Gao, Pei-Chun Liao, Bing-Hong Huang, Tao You, You-Yang Zhang, and Jun-Qing Li. Historical biogeography of Acer L.(Sapindaceae): genetic evidence for Out-of-Asia hypothesis with multiple dispersals to North America and Europe. Dec. 03, 2020. Scientific Reports 10, Article number 21178 (2020).
Goby Walnut and Western Hardwoods. 12/03/18. Let’s Talk about Figure.
Lentink, D. et al. Leading-Edge Vortices Elevate Lift of Autorotating Plant Seeds. Science. 12 June 2009. Vol. 324, Issue 5933. Pp. 1438-1440.
Nadkarni, N. M. 1984. Biomass and mineral capital of epiphytes in an Acer macrophyllum community of a temperate moist coniferous forest, Olympic Peninsula, Washington State. Canadian Journal of Botany, Volume 62, Number 11. Pages 2223-22228.
Nichols, Nhatt. 6/1/2023. Sugaring the Pacific Northwest: How climate and cost cramp bigleaf maple syrup production.
Niemiec, Stanley S. et al.1995. Hardwoods of the Pacific Northwest. Oregon State University, Forest Research Laboratory.
Rose, Ian. Solving the Mystery of Washington’s Bigleaf Maple Die-off. Earth Island Journal. January 19, 2022.
Sedler, Moses. 2/1/21. Anatomy of a Violin—What a Violin is Made of.
Sequin, Margareta. 2012. The Chemistry of Plants: Perfumes, Pigments, and Poisons.
Smith College. Stradivarius Violins. Climate in Arts and History: Promoting Climate Literacy Across Disciplines.
Snohomish Conservation District. 3/21/22. Sweet Secrets of Bigleaf Maple.
Sudworth, George B. 1967. Forest Trees of the Pacific Slope.
Tejo, Camila F. et al. 2014. Canopy Soils of Sitka Spruce and Bigleaf Maple in the Queets River Watershed, Washington. Soil Science Society of America Journal 78 (S1):S118.
All photos by Laura Baker except where otherwise credited. Photos 4 and 5 courtesy of California Big Trees, a project of the Urban Forest Ecosystems Institute at California Polytechnic University at San Luis Obispo. Photos 37 and 38 courtesy of Smithsonian Institution. Thanks to William Ericson for the identification of the insects in Photos 18 and 19.